Inside Quantum Chip Manufacturing: From Qubits to Fabrication
2025.05.12 · Blog
Introduction: What Is Quantum Chip Manufacturing?
Quantum chip manufacturing is the process of designing, fabricating, and packaging chips that operate on the principles of quantum mechanics. These chips lie at the heart of quantum computers and are responsible for generating and manipulating qubits—the quantum equivalent of classical bits.
Unlike classical chips, quantum chips must preserve quantum states, making their manufacturing significantly more complex and interdisciplinary.
Key Components of a Quantum Chip
Before diving into the manufacturing process, it's important to understand what makes up a quantum chip:
-
Qubits: The basic unit of quantum information. Different technologies use different qubit types, such as superconducting circuits, trapped ions, or spin-based systems.
-
Quantum Gates: Circuit elements that manipulate qubits through operations like entanglement and superposition.
-
Cryogenic Infrastructure: Most quantum chips operate at near absolute zero, requiring specialized cryostats and thermal shielding.
Step-by-Step Guide: How Quantum Chips Are Manufactured
Quantum chips lie at the heart of every quantum computer. Their fabrication demands expertise across physics, materials science, electrical engineering, and nanotechnology.
Here's a step-by-step guide that explains how quantum chips are manufactured—from theoretical design to cryogenic testing and final quantum system integration.
Step 1: Qubit Technology Selection, Design, and Simulation
The process begins with selecting the appropriate qubit technology. Common types include:
-
Superconducting qubits (e.g., based on Josephson junctions)
-
Trapped ions
-
Quantum dots
-
Spin qubits
-
Neutral atoms
Superconducting qubits are the most widely used due to their scalability and compatibility with existing semiconductor processes and high-speed gate operations.
Once qubit technology is selected, engineers simulate and model qubit behavior using quantum mechanical principles. Parameters such as coherence time, gate fidelity, crosstalk, and scalability are tested digitally and optimized before physical fabrication begins.
Note: Design software is used to simulate quantum circuits, resonator couplings, and control/readout schemes.
Step 2: Material Selection
The next step involves choosing suitable materials. For superconducting qubits, commonly used materials include:
-
Aluminum or niobium (superconducting metals)
-
Silicon or sapphire (wafer substrates)
These materials must exhibit ultra-low defect densities, stable superconductivity, and low microwave loss at cryogenic temperatures.
Note: Material purity directly affects qubit performance and coherence time.
Step 3: Nanofabrication and Lithography
Quantum chips are fabricated in cleanrooms using advanced nanofabrication techniques:
-
Electron-beam lithography for ultra-fine patterning
-
Atomic layer deposition (ALD) for thin films
-
Etching to remove unnecessary materials
-
Lift-off processes to define Josephson junctions and wiring
Precision at the nanometer scale is crucial—minor defects can degrade performance or completely disrupt qubit operation.
Note: Josephson junctions, resonators, and microwave lines are formed in this step.
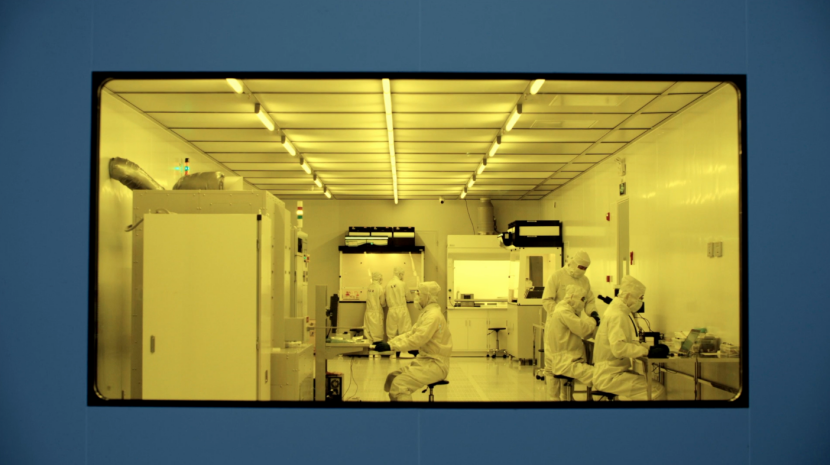
Step 4: Layering, Etching, and Assembly
After patterning, multilayered structures are built using:
-
Thin-film deposition for control and readout lines
-
Reactive ion etching to sculpt complex 3D geometries
-
Surface passivation to prevent charge noise and oxidation
Each layer must align precisely with nanometer accuracy to ensure proper functioning of qubits and coupling structures.
Note: At this scale, even a single dust particle can compromise an entire chip. These steps must be performed with extreme precision to prevent any defects that could introduce decoherence.
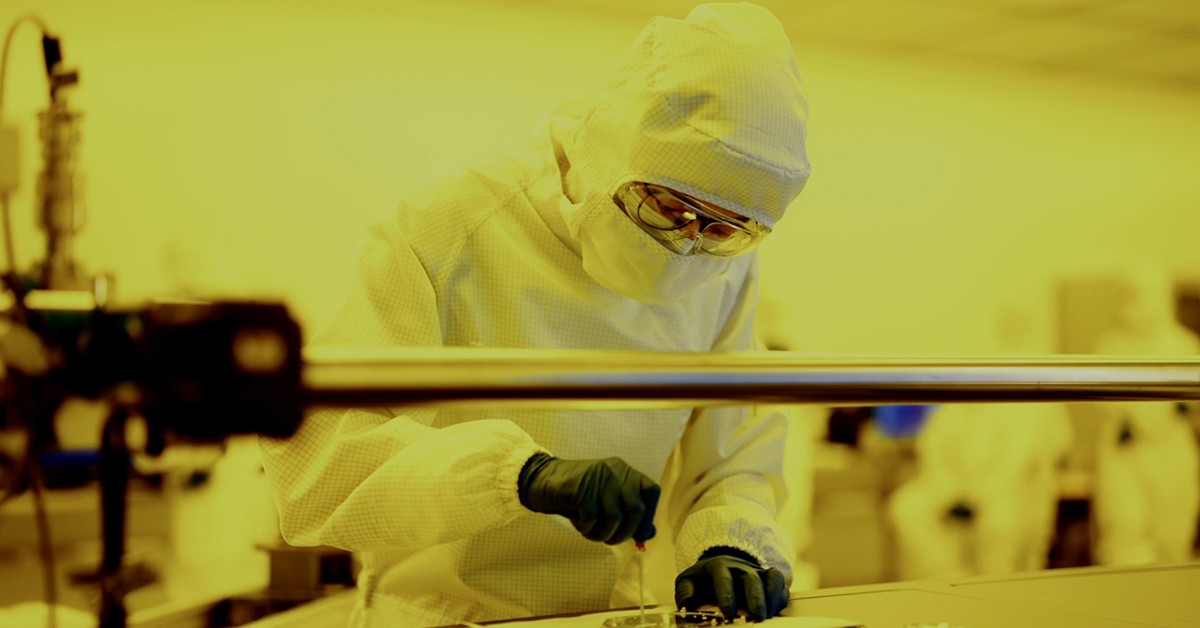
Step 5: Packaging and Cryogenic Integration
Once quantum chip manufacturing is complete, the chip undergoes packaging:
-
Mounted onto a microwave-compatible chip carrier
-
Connected via ultra-low-loss coaxial cables
-
Shielded using magnetic and thermal enclosures
To operate, quantum chips must be cooled to millikelvin temperatures, near absolute zero, using dilution refrigerators. This ensures stable quantum states and minimizes decoherence.
Note: Thermal, magnetic, and vibrational isolation is crucial at this stage.
Step 6: Testing, Calibration, and Characterization
Chips are then tested inside cryostats with high-frequency electronics:
-
Coherence time (T1/T2) measurements
-
Gate fidelity benchmarks (using randomized benchmarking)
-
Qubit-qubit crosstalk analysis
-
Error rate tracking under repeated operations
If results meet performance criteria, the chip is deemed ready for system-level integration.
Note: Qubits that fail this phase are often recharacterized, retuned, or discarded.
Step 7: Integration Into Quantum Computing Systems
In the final step, validated chips are integrated with:
-
Quantum control systems (microwave generators, FPGAs, AWGs)
-
Classical computers for readout and error correction
-
Software stacks for algorithm execution
These systems are then deployed in:
-
Quantum research labs
-
Quantum algorithm development
-
Cloud-based quantum platforms
-
Commercial applications in finance, materials, AI, etc.
Note: Full-stack quantum computers require precise hardware-software co-design.
Curious how superconducting quantum chips are fabricated? Watch this YouTube video to explore the fascinating process behind quantum chip manufacturing!
Recommended Articles:
Challenges in Quantum Chip Manufacturing
-
Extreme Sensitivity: Even tiny amounts of noise or impurities can destroy quantum coherence.
-
Low Yields: Due to complexity and sensitivity, many chips fail quality standards.
-
Scalability: Building chips with hundreds or thousands of qubits is still a major hurdle.
Future Outlook: Toward Scalable Quantum Chip Manufacturing
Top quantum computing companies like IBM, Google, Intel, and SpinQ are investing heavily in developing scalable quantum chip manufacturing pipelines. Innovations in cryo-CMOS control electronics, 3D integration, and fault-tolerant architectures are key to achieving large-scale, practical quantum computing.
As quantum chips transition from lab prototypes to commercial hardware, the focus will shift toward automation, reliability, and miniaturization—much like the evolution of classical semiconductor chips.
Conclusion
Quantum chip manufacturing is a complex yet fascinating process that sits at the intersection of physics, materials science, and nanotechnology. With ongoing breakthroughs, the industry is steadily moving closer to realizing large-scale, fault-tolerant quantum computers. Understanding how these chips are built is essential for anyone following the future of computing.
Featured Content